Amyloid Hypothesis vs Tau Hypothesis
*updated from an original article posted August 2018
Plaques, Tangles, and the Pathology of Alzheimer’s Disease
Alzheimer’s Disease (AD) is a neurodegenerative disease that is becoming increasingly common as the global population ages. The search for a cure has yet to come to fruition, but over a century of research has allowed scientists to better understand how the disease progresses and what contributes to its development. Brains with AD contain two main features: plaques consisting of a peptide called amyloid-beta and neurofibrillary tangles consisting of a protein called tau. Amyloid-beta and tau aggregation are both thought to contribute to AD, but there are different hypotheses as to which aggregates first, which should be the target for treatments, and how the two proteins interact.
A Brief History of Alzheimer’s Disease Research
When Alois Alzheimer documented “presenile dementia” in his 51-year-old dementia patient Auguste Deter, he noticed short-term memory loss and delusions that were common in patients in their 70s, but not in their 50s. After her death, he observed anomalies in her brain: plaques and tangles.1 Although plaques had been previously seen in other dementia cases,2 Alzheimer was the first to describe the tangles. His senior colleague, Emil Kraepelin, suggested he had discovered a new disease and named it Alzheimer’s Disease. Cases of autosomal dominant, or early-onset familial AD, which are inherited from a parent with an abnormal gene and occur in patients under the age of 65, were documented and studied in the 1930s and 40s. This was believed to be a different disease than “senile dementia,” which had similar symptoms but occurred in patients over the age of 65 and did not follow an inherited autosomal dominant inheritance pattern.3
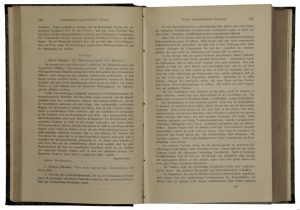
“Über eine eigenartige Erkrankung der Hirnrinde,”which translates to “About a Peculiar Disease of the Cerebral Cortex.” The original case study by Alois Alzheimer was published in 1907. By © Foto H.-P.Haack [CC BY 3.0 (https://creativecommons.org/licenses/by/3.0)], via Wikimedia Commons
The Amyloid Hypothesis
Individuals with Down syndrome can often experience neurological changes and memory loss later in life. This includes formation of plaques similar to those seen in AD patients.5 In 1984, Glenner and Wong isolated a 42 kDa peptide called amyloid-β (Aβ) from the plaques of both AD and Down syndrome brains.6 They proposed that because AD and Down syndrome plaques both contain Aβ, the conditions share a common pathogenic process. Furthermore, they claimed that since Aβ is encoded in chromosome 21, and Down syndrome is a result of the trisomy of chromosome 21, the genes on chromosome 21 are responsible for AD pathology.6 This led to the amyloid hypothesis (also known as the amyloid-beta hypothesis or amyloid cascade hypothesis), which states that Aβ aggregation triggers the cascade of events that ultimately lead to AD pathology and symptoms.7 This cascade of events includes the aggregation of hyperphosphorylated tau proteins into neurofibrillary tangles (NFTs), as well as inflammation and oxidative stress causing neuronal dysfunction and death.
At this time, there were numerous complex and conflicting theories regarding the pathogenesis of AD.7 The Down syndrome link was compelling, and the amyloid hypothesis was relatively simple and provided researchers with a therapeutic target.7 This made it the focus of extensive research over the next several decades.
Evidence for the Amyloid Hypothesis
Alzheimer’s Genes and the Amyloid Hypothesis
The APP gene is located on chromosome 21 and encodes the Amyloid Precursor Protein (APP). The amyloid fragment is generated when APP is cleaved by gamma-secretase. The component of gamma-secretase that cleaves APP consists of presenilin transmembrane proteins. Mutations in the APP gene as well as the PSEN genes that encode presenilin have been linked to early-onset AD.8 These mutations favour the formation of longer amyloid fragments that clump together into oligomers and fibrils more readily.
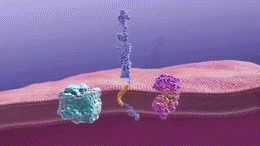
Gamma-secretase cleaves the amyloid precursor protein to generate beta-amyloid fragments. These fragments then oligomerize. Source: National Institute on Aging [Public domain]
The Role of Amyloid Oligomers in Alzheimer’s Disease
While the original amyloid hypothesis postulated that Aβ plaques are the toxic species that kill neurons, more recent research suggests that amyloid oligomers, rather than plaques, are neurotoxic.11 Injecting Aβ oligomers into the hippocampi of mice induces AD pathology12 and oligomers interact with neurons and glial cells to induce inflammation, oxidative stress, and tau phosphorylation.9
Alzheimer’s Treatments Targeting Amyloid Beta
Crenezumab is a monoclonal antibody that binds to Aβ monomers and oligomers. Although it did not meet the specified endpoints in a phase II trial, it was generally well tolerated and outperformed the placebo when high doses were given to patients with very mild AD.13 However, two Phase III trials were halted when interim data showed crezenumab was unlikely to meet primary endpoints. If Aβ accumulation was the initial event that triggered neurodegeneration, then preventing this accumulation would prevent AD. Some possible approaches to address Aβ aggregation are passive immunization, where manufactured monoclonal antibodies are administered to patients, and active immunity, where people are vaccinated so they produce their own antibodies against Aβ. In any event, these antibodies should bind to Aβ proteins and prevent them from aggregating into plaques.
Aducanumab is another monoclonal antibody that targets aggregated Aβ. It was shown to reduce Aβ and slow cognitive decline in phase II trials. Phase III trials were halted after they were also determined to be unlikely to meet their primary endpoints14 but Biogen now plans to file for FDA approval after analysis of a larger data set showed promising results for patients with early-stage AD who were given high doses of the drug.
AN1792, a vaccine against Aβ, was discontinued after 6% of participants developed meningoencephalitis (inflammation of the meninges and brain). It produced very few antibody responders and showed no significance between antibody responder and placebo groups after 12 months.15 However, a follow-up study showed that antibody responders experienced significantly less cognitive decline than placebo recipients.16
It is possible that in order to prevent or cure AD, treatment must be given before symptoms are shown. Failure of certain drugs to meet clinical endpoints could mean that they target the wrong biomarker, but it could also indicate that they are being administered too late to have a significant effect. Even if amyloid plaques are cleared, the damage to the brain may not be repaired. In the words of John Hardy, a neurogeneticist at University College London, “The general view is these are the right drugs, but they’re too late. It’s like taking statins when you’re having a heart attack.”17 However, Aβ-targeted therapies have been largely unsuccessful, leading scientists to become increasingly skeptical of the amyloid hypothesis and explore other potential pathogeneses of AD.
Evidence against the Amyloid Hypothesis
Solanezumab, bapineuzumab, and gantenerumab, antibodies targeting Aβ, all failed to reduce cognitive decline in phase III clinical trials.18,19,20 Ponezumab, another antibody, was abandoned after phase II.21
While there are several possible reasons Aβ antibodies have failed to effectively treat AD, one possibility that cannot be ignored is that Aβ accumulation does not cause neurodegeneration. Aβ plaques occur in cognitively normal individuals and could be a sign of normal aging.7 Individuals with Down syndrome can develop Aβ plaques as adolescents without developing AD later in life.22 Furthermore, dementia severity correlates more strongly with NFTs than with Aβ plaques.23
The roles of APP and Aβ remain unclear. Following traumatic brain injury (TBI), APP is upregulated and offers neuroprotective effects including improved motor and cognitive outcomes.24 This suggests that the production and aggregation of APP and Aβ may be responses to neurological damage rather than the root causes of neurodegeneration.
While the amyloid hypothesis has yet to produce an effective treatment for AD, it does not necessarily indicate that Aβ has no involvement in the development of the disease. It does, however, present the possibility that Aβ accumulation does not trigger the cascade that leads to neurodegeneration, and that other possibilities should be considered.
The Tau Hypothesis
In 1986, Kosik et al. discovered that NFTs were composed of phosphorylated tau proteins.25 Microtubule-Associated Protein Tau (MAPT) stabilizes microtubules26 and can undergo a variety of post-translational modifications including phosphorylation. When tau is hyperphosphorylated, it dissociates from microtubules and aggregates into paired helical filaments (PHFs) and NFTs. The tau hypothesis postulates that tau tangle pathology precedes Aβ plaque formation and that tau phosphorylation and aggregation is the primary cause of neurodegeneration in AD.
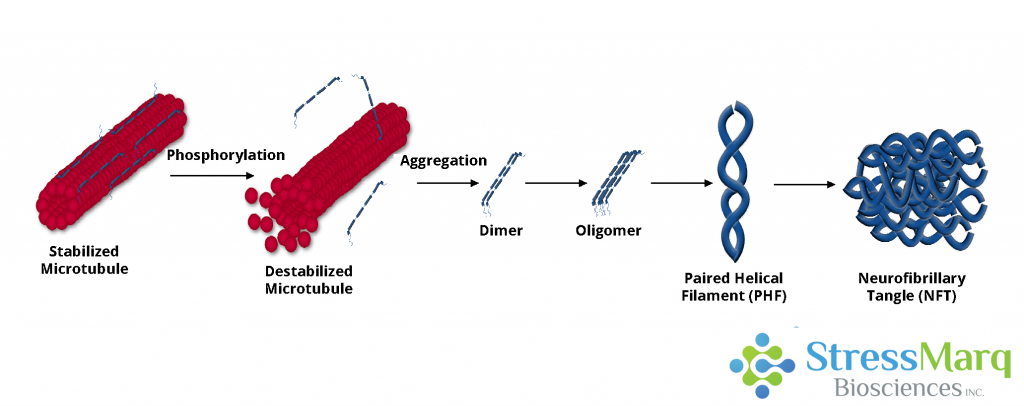
Tau dissociates from microtubules, leading to their destabilization. It then aggregates into oligomers, paired helical filaments, and ultimately neurofibrillary tangles.
Evidence for the Tau Hypothesis
Tau phosphorylation reduces its ability to promote microtubule assembly,27 causing neurodegeneration via synaptic dysfunction and neuronal loss.28 Neurofibrillary tangles can also cause neuronal dysfunction and death. While the amyloid hypothesis suggests that tau aggregation occurs downstream of Aβ aggregation, tau tangles can be found in the brains of patients with very mild dementia and no Aβ pathology.9 Tau pathology also correlates more closely with AD progression and severity than Aβ plaque load does.27
While Aβ plaques can occur in individuals who do not experience neurodegeneration, the same cannot be said for NFTs, which are seen in frontotemporal dementia and other tauopathies. This may be because amyloid plaques are located in the extracellular space whereas tau tangles occur within neurons where they can severely impair axonal transport.
Decades of focus on the amyloid hypothesis at the expense of the tau hypothesis means that generally, tau research is at an earlier stage.29 However, tau-based strategies have shown some promising results29 and there are currently seven anti-tau therapies in phase II trials.30
Evidence Against the Tau Hypothesis
Unfortunately, many anti-tau therapies have also failed in clinical trials. Glycogen synthase kinase 3 beta (GSK-3β) is a protein kinase that facilitates tau phosphorylation, and is therefore an attractive target for anti-tau therapies. However, Tideglusib, a GSK-3β inhibitor, did not show significant clinical benefit in a phase II trial.31
Methylene blue dye derivatives Trx0014 and LMTM inhibit tau aggregation and appeared to slow cognitive decline in phase III trials, but the methodology and claims of efficacy remain controversial.29
A Joint Mechanism
The tau and amyloid hypotheses are perceived as competing, pitting “Tauists” against “βAptists.” However, AD is multifaceted and will likely require a multifaceted approach to treatment. There are numerous proposed theories about how Aβ and tau interact to induce AD pathology: metaphors include a toxic pas de deux,32 a trigger and a bullet,33 and a match that starts bush fires.17 Some researchers believe amyloid and tau follow independent but parallel mechanisms,34 but most propose that Aβ promotes the formation of tau tangles, which then damage neurons.17 There are many proposed mechanisms including Aβ-induced inflammation or synaptic defects promoting tau aggregation and Aβ cross-seeding of tau.35 There is also the possibility of a feedback loop where tau mediates Aβ toxicity.32
Other Possible Mechanisms
Amyloid plaques and tau tangles are not the only features seen in the AD brain; oxidative stress and inflammation also occur. The mitochondrial cascade hypothesis suggests that impaired mitochondrial function, which, like AD, has a strong maternal genetic contribution, initiates tau and Aβ aggregation downstream.36 Herpes simplex virus type 1 (HSV1) has been associated with an increased risk of AD in APOE-ε4 carriers, meaning viruses and other microbes may play a role in the disease.37
Acetylcholine (ACh) is a neurotransmitter released by motor neurons to activate muscles. It decreases in concentration and its function is impaired in AD patients.38 The cholinergic hypothesis postulates that the cognitive impairment seen in AD is caused by the lack of ACh. Four acetylcholinesterase inhibitors are currently used to treat AD, and work by inhibiting the enzymes that break down acetylcholine. These medications treat symptoms, but do not cure AD. However, ACh dysfunction may contribute to AD by inducing inflammation and Aβ and tau aggregation.39
Alzheimer’s pathology is complex, but the past century has seen significant advancement in our understanding of the disease and its causes. A cure may not be as simple as researchers had hoped; it may be prophylactic or require a multi-pronged approach. Bill Gates recently donated $100 million to AD research, and advancements are being made in labs around the world.
StressMarq offers Aβ monomers, oligomers and pre-formed fibrils, and also a range of tau fibrilized proteins and a variety of Aβ and tau antibodies. Our strategic efforts are currently focused on being a world leader in the development and supply of active, pathology-inducing fibrilized proteins (and their derivatives), protein oligomers, and antibodies to these constructs to assist researchers studying Alzheimer’s, Parkinson’s, and other neurodegenerative diseases.
References
- Alzheimer, A. Z. Ueber eine eigenartige Erkrankung der Hirnrinde. Allg Z Psych-Gerichtl. Med. 64, 146–148 (1907)
- Redlich, F. Uber miliare Sklerose der Hirnrinde bei seniler Atrophie. Jarbucher fur Psychiatrie und Neurologie, 1898 (1898), p. 17
- Hardy, J. A Hundred Years of Alzheimer’s Disease Research. Neuron. 52(1):3-13. (2006).
- Tomlinson, B.E., Blessed G., Roth, M. Observations on the brains of demented old people. Neurol. Sci., 11 (1970), pp. 205-242
- Mann DM. Histopathology. 1988;13(2):125–137
- Glenner, G., Wong, C. Alzheimer’s disease and Down’s syndrome: Sharing of a unique cerebrovascular amyloid fibril protein. Bioc & Biophys Res Comm. 122(6):1131-1135. (1984).
- Morris et al. Inconsistencies and Controversies Surrounding the Amyloid Hypothesis of Alzheimer’s Disease. Acta Neuropathologica Communications 2014, 2:135.
- Boeras DI, Granic A, Padmanabhan J, Crespo NC, Rojiani AM, Potter H. Alzheimer’s presenilin 1 causes chromosome missegregation and aneuploidy. Neurobiol Aging. 2008 Mar; 29(3):319-28.
- Jesus R. de Paula, V., Guimaraes, F.B., Diniz, B.S., Forlenza, O.V. Neurobiological pathways to Alzheimer’s disease: Amyloid-beta, TAU protein, or both? Dement Neuropsychol. 2009 Jul-Sep; 3(3): 188–194.
- Kanekiyo, T., Xu, H., Bu, G. ApoE and Aβ in Alzheimer’s disease: accidental encounters or partners? Neuron. (2014). 81(4): 740-754.
- Kim, J., Basak, J.M., Holtzman, D. The Role of Apolipoprotein E in Alzheimer’s Disease. Neuron. 2009; 63(3): 287-303.
- Brouillette J, Caillierez R, Zommer N, Alves-Pires C, Benilova I, Blum D, De Strooper B, Buée L (2012) Neurotoxicity and Memory Deficits Induced by Soluble Low-Molecular-Weight Amyloid-β1–42 Oligomers Are Revealed In Vivo by Using a Novel Animal Model. J Neurosci. 32:7852–7861
- Cummings, J.L. et al. A phase 2 randomized trial of crenezumab in mild to moderate Alzheimer disease. Neurology® 2018;0:e1-e9
- investors.biogen.com/news-releases/news-release-details/eisai-and-biogen-announce-positive-topline-results-final
- Gilman, S. et al. Clinical effects of Aβ immunization (AN1792) in patients with AD in an interrupted trial. Neurology. (2005). 64(9).
- Vellas, B. et al. Long-term follow-up of patients immunized with AN1792: reduced functional decline in antibody responders. Curr Alzheimer Res. (2009). 6(2): 144-51.
- nature.com/articles/d41586-018-05719-4
- Honig, L. S. et al. Trial of Solanezumab for Mild Dementia Due to Alzheimer’s Disease. N Engl J Med 2018; 378:321-330.
- Salloway, S. et al. Two Phase 3 Trials of Bapineuzumab in Mild-to-Moderate Alzheimer’s Disease. N Engl J Med 2014; 370:322-333.
- Rygiel, K. Novel strategies for Alzheimer’s disease treatment: An overview of anti-amyloid beta monoclonal antibodies. Indian J Pharmacol. (2016). 48(6): 629-636.
- Van Dyck, C.H. Anti-Amyloid-β Monoclonal Antibodies for Alzheimer’s Disease: Pitfalls and Promise. Biol Psychiatry. (2018). 83(4): 311-319.
- Zigman WB, Devenny DA, Krinsky-McHale SJ, Jenkins EC, Urv TK, Wegiel J, Schupf N, Silverman W (2008) Alzheimer’s Disease in Adults with Down Syndrome. Int Rev Res Mental Retardation 36:103–145.
- Nelson, P.T. et al. Correlation of Alzheimer Disease Neuropathologic Changes with Cognitive Status: A Review of the Literature. J Neuropathol Exp Neurol. (2012). 71(5): 362-381.
- Plummer, S. et al. The Neuroprotective Properties of the Amyloid Precursor Protein Following Traumatic Brain Injury. Aging Dis. (2016). 7(2): 163-179.
- Kosik, K.S, Joachim, C.L, and Selkoe, D.J. Microtubule-associated protein tau (tau) is a major antigenic component of paired helical filaments in Alzheimer disease. Proc Natl Acad Sci USA. (1986). 83(11): 4044-4048.
- Lindwall G, Cole RD. The purification of tau protein and the occurrence of two phosphorylation states of tau in brain. J Biol Chem. 1984 Oct 10;259(19):12241–12245.
- Braak, H. & Braak, E. Neuropathological stageing of Alzheimer-related changes. Acta Neuropathol. (1991). 82(4):239-59.
- Di, J. et al. Abnormal tau induces cognitive impairment through two different mechanisms: synaptic dysfunction and neuronal loss. Scientific Reports, 6 (2016).
- Medina, M. An Overview on the Clinical Development of Tau-Based Therapeutics. Int J Mol Sci. (2018). 19(4):1160.
- Cummings, J. et al. Alzheimer’s disease drug development pipeline: 2018. Alz & Dem: Transl Res & Clin Interventions. (2018). 4:195-214.
- Lovestone S, Boada M, Dubois B, Hüll M, Rinne JO, Huppertz HJ, Calero M, Andrés MV, Gómez-Carrillo B, León T, del Ser T, ARGO investigators. A phase II trial of tideglusib in Alzheimer’s disease. J Alzheimers Dis. 2015; 45(1):75-88.
- Ittner, L. M. & Gotz, J. Amyloid-β and tau – a toxic pas de deux in Alzheimer’s disease. Nat Rev Neurosci. (2011). (12):67-72.
- Bloom, G.S. Amyloid-β and tau: the trigger and bullet in Alzheimer disease pathogenesis. JAMA Neurol. (2014). 71(4):505-8.
- Small, S.A., & Duff, K. Linking Aβ and Tau in Late-Onset Alzheimer’s Disease: A Dual Pathway Hypothesis. Neuron. (2008). 60(4): 534-542.
- Stancu, I., Vasconcelos, B., Terwel, D., & Dewachter, I. Models of β-Amyloid induced Tau-pathology: the long and “folded” road to understand the mechanism. Mol Neurodegener. (2014). 9:51.
- Swerdlow, R. et al. The Alzheimer’s Disease Mitochondrial Cascade Hypothesis. Journal of Alzheimer’s Disease. (2010). 29:S265-S279.
- Itzhaki, R.F. et al. Herpes simplex virus type 1 in brain and risk of Alzheimer’s disease. Lancet. (1997). 349(9047):241-4.
- Francis, P.T. et al. The interplay of neurotransmitters in Alzheimer’s disease. CNS Spectr. (2005). 10(11 Suppl 18):6-9.
- Shen ZX (2004). “Brain cholinesterases: II. The molecular and cellular basis of Alzheimer’s disease”. Hypotheses. 63 (2): 308–21.
Leave a Reply